Search Constraints
1 - 5 of 5
Number of results to display per page
View results as:
Search Results
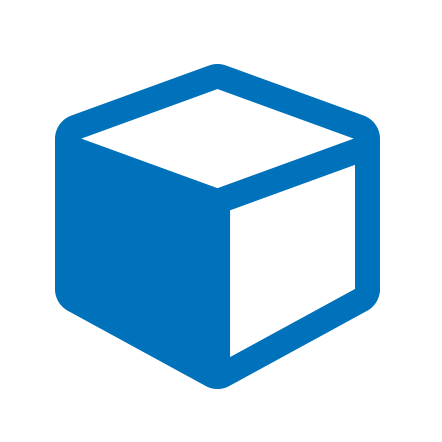
- Creator:
- Luyet, Chloe, Elvati, Paolo, Vinh, Jordan, and Violi, Angela
- Description:
- A growing body of work has linked key biological activities to the mechanical properties of cellular membranes, and as a means of identification. Here, we present a computational approach to simulate and compare the vibrational spectra in the low-THz region for mammalian and bacterial membranes, investigating the effect of membrane asymmetry and composition, as well as the conserved frequencies of a specific cell. We find that asymmetry does not impact the vibrational spectra, and the impact of sterols depends on the mobility of the components of the membrane. We demonstrate that vibrational spectra can be used to distinguish between membranes and, therefore, could be used in identification of different organisms. The method presented, here, can be immediately extended to other biological structures (e.g., amyloid fibers, polysaccharides, and protein-ligand structures) in order to fingerprint and understand vibrations of numerous biologically-relevant nanoscale structures.
- Keyword:
- molecular dynamics, membranes, mechanical vibration, bacterial identification, and Staphylococcus aureus
- Citation to related publication:
- Luyet C, Elvati P, Vinh J, Violi A. Low-THz Vibrations of Biological Membranes. Membranes. 2023; 13(2):139. https://doi.org/10.3390/membranes13020139
- Discipline:
- Engineering
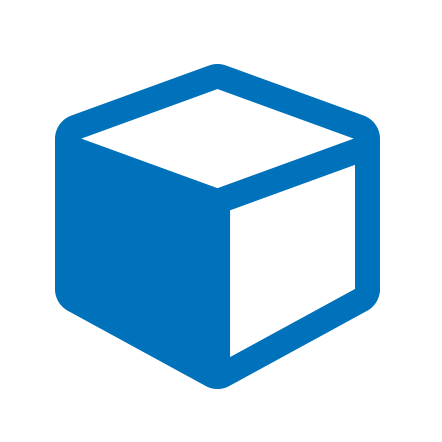
- Creator:
- Elvati, Paolo, Luyet, Chloe, Wang, Yichun, Liu, Changjiang, VanEpps, J. Scott, Kotov, Nicholas A., and Violi, Angela
- Description:
- Amyloid nanofibers are abundant in microorganisms and are integral components of many biofilms, serving various purposes, from virulent to structural. Nonetheless, the precise characterization of bacterial amyloid nanofibers has been elusive, with incomplete and contradicting results. The present work focuses on the molecular details and characteristics of PSMa1-derived functional amyloids present in Staphylococcus aureus biofilms, using a combination of computational and experimental techniques, to develop a model that can aid the design of compounds to control amyloid formation. Results from molecular dynamics simulations, guided and supported by spectroscopy and microscopy, show that PSMa1 amyloid nanofibers present a helical structure formed by two protofilaments, have an average diameter of about 12 nm, and adopt a left-handed helicity with a periodicity of approximately 72 nm. The chirality of the self-assembled nanofibers, an intrinsic geometric property of its constituent peptides, is central to determining the fibers' lateral growth.
- Keyword:
- molecular self-assembly, computational nanotechnology, nanobiotechnology, and structural properties
- Citation to related publication:
- Paolo Elvati, Chloe Luyet, Yichun Wang, Changjiang Liu, J. Scott VanEpps, Nicholas A. Kotov, and Angela Violi ACS Applied Nano Materials 2023 6 (8), 6594-6604 DOI: 10.1021/acsanm.3c00174
- Discipline:
- Engineering and Science
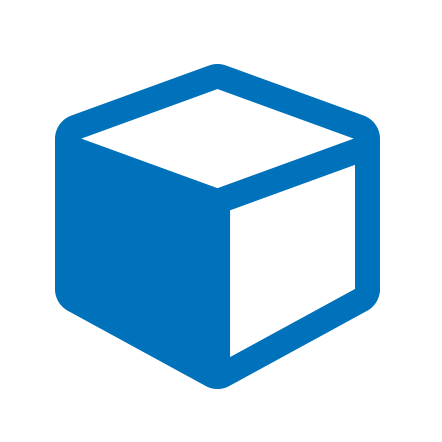
3. Supporting data: Domain-agnostic predictions of nanoscale interactions in proteins and nanoparticles
- Creator:
- Saldinger, Jacob, Raymond, Matt , Elvati, Paolo, and Violi, Angela
- Description:
- The accurate and rapid prediction of generic nanoscale interactions is a challenging problem with broad applications. Much of biology functions at the nanoscale, and our ability to manipulate materials and purposefully engage biological machinery requires knowledge of nano-bio interfaces. While several protein-protein interaction models are available, they leverage protein-specific information, limiting their abstraction to other structures. Here, we present NeCLAS, a general, and rapid machine learning pipeline that predicts the location of nanoscale interactions, providing human-intelligible predictions. Two key aspects distinguish NeCLAS: coarse-grained representations, and the use of environmental features to encode the chemical neighborhood. We showcase NeCLAS with challenges for protein-protein, protein-nanoparticle and nanoparticle-nanoparticle systems, demonstrating that NeCLAS replicates computationally- and experimentally-observed interactions. NeCLAS outperforms current nanoscale prediction models, and it shows cross-domain validity, qualifying as a tool for basic research, rapid prototyping, and design of nanostructures., Software: - To reproduce all-atom molecular dynamics (MD) NAMD is required (version 2.14 or later is suggested). NAMD software and documentation can be found at https://www.ks.uiuc.edu/Research/namd/, - To reproduce coarse-grained MD simulations, LAMMPS (version 29 Sep 2021 - Update 2 or later is suggested). LAMMPS software and documentation can be found at https://www.lammps.org, - To rebuild free energy profiles, the PLUMED plugin (version 2.6) was used. PLUMED software and documentation can be found at https://www.plumed.org/ , and - To generate force matching potentials, the was used the OpenMSCG software was used. OpenMSCG software and documentation can be found at https://software.rcc.uchicago.edu/mscg/
- Keyword:
- Neural Networks, Proteins, Dimensionality Reduction, Nanoparticles, and Coarse-Graining
- Citation to related publication:
- https://www.biorxiv.org/content/10.1101/2022.08.09.503361v2
- Discipline:
- Science
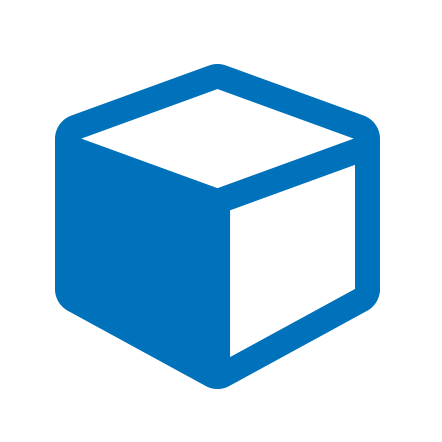
4. On the growth of Si nanoparticles in non-thermal plasma: physisorption to chemisorption conversion
- Creator:
- Shi, Xuetao, Elvati, Paolo, and Violi, Angela
- Description:
- Non-thermal plasma systems offer unique opportunities in the fields of bio-imaging, drug delivery, photovoltaics, microelectronics manufacturing. Such interests are largely inspired by the fact that hot plasma electrons coexist with neutral species and ions close to room-temperature under non-thermal plasma conditions. Modeling of these systems requires a deep understanding of the atomistic processes underlying the rich chemistry of the various radicals and ions with the nascent nanoparticle surface. A key parameter for determining the contribution of a certain radical/ion species to the nanoparticle surface growth, called sticking coefficient, is computed as a weighted sum from the simulated sticking outcomes with different collision velocities drawn from a Maxwell-Boltzmann distribution at certain temperatures. In this work, the collisions of SiHx (x=1-4) fragments and silicon cluster (Si4, Si2H6, and Si29H36) surfaces, responsible for the sticking coefficients, are simulated by molecular dynamics (MD) with a reactive force field. The dependence of sticking coefficients on temperature, H coverage of both silane fragments and cluster surfaces, and the size of the cluster, are systematically examined. And the mechanism underlying the sticking events, specifically the conversion of physical aggregation to chemisorption is investigated to better understand the complex interplay between factors influencing the surface growth. The detailed and multi-parameter model of sticking coefficients, accompanied by the mechanism study of physisorption to chemisorption conversion, provides a more accurate and robust approximation of surface growth rate using sticking coefficients, and a deeper understanding of surface growth processes, for the wider non-thermal plasma simulation community.
- Keyword:
- Sticking coefficients, Silanes, Molecular Dynamics, Non-equilibrium, and Aggregation mechanisms
- Citation to related publication:
- Shi, X., Elvati, P., Violi, A. (2021). On the growth of Si nanoparticles in non-thermal plasma: physisorption to chemisorption conversion. J. Phys. D. Submitted.
- Discipline:
- Science
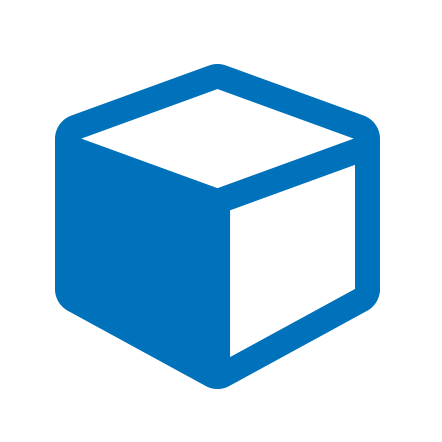
- Creator:
- Wang, Qi, Elvati, Paolo, Kim, Doohyun, Johansson, K. Olof, Schrader, Paul E., Michelsen, Hope A., and Violi, Angela
- Description:
- Datasets for article in CARBON: Spatial dependence of the growth of polycyclic aromatic compounds in an ethylene counterflow flame. The experiment VUV-AMS measurements ("VUV_AMS_C2H4_Counterflow.txt") consists aerosol mass spectra data from an atmospheric-pressure ethylene/oxygen/argon counterflow diffusion flame described in Johansson et al., Proc. Combust. Inst. 36, 799-806 (2017) doi:10.1016/j.proci.2016.07.130., The experiment VUV-MBMS measurements ("VUV_MBMS_C2H4_Counterflow.txt") consists gas-phase data from an atmospheric-pressure ethylene/oxygen/argon counterflow diffusion flame described in Johansson et al., Proc. Combust. Inst. 36, 799-806 (2017) doi:10.1016/j.proci.2016.07.130., 2D CFD simulation results by KAUST mechanism II ("CFD_KM2_results.xlsx") consists stabilized CFD gas-phase species profiles along different x,y,z coordinates. Species are given by mole fractions., The SNapS2 simulation results ("SNapS2_results.zip") consist streamline I (from fuel side), i (from oxidizer side), and middle (DFFO = 5.0mm) for producing results in Fig. 5, Fig. 6, and Table 1. Three folders under each streamline ("C5H6", "C6H5CH3", and "C6H6") represent simulations by using different seeds (cyclopentadiene, toluene, and benzene respectively). The text files inside each folder are a single trace (time-history) for one SNapS2 simulation. Text file name consists "starting time"+"."+"simulation number"+".txt". For example 0.041.25.txt meaning the 25th simulation starting at 0.041s. Four columns inside the text files represent time, molecular mass, reaction index, and SMILES (Simplified molecular-input line-entry system) of the molecule., and Data citation: Wang, Q., Elvati, P., Kim, D., Johansson, K.O., Schrader, P.E., Michelsen, H.A., Violi, A. (2019). Spatial dependence of the growth of polycyclic aromatic compounds in an ethylene counterflow flame: experimental measurements and simulation results [Data set]. University of Michigan Deep Blue Data Repository. https://doi.org/10.7302/69e6-cd20
- Citation to related publication:
- Wang, Q., Elvati, P., Kim, D., Johansson, K.O., Schrader, P.E., Michelsen, H.A., Violi, A., 2019. Spatial dependence of the growth of polycyclic aromatic compounds in an ethylene counterflow flame. Carbon 149, 328–335. https://doi.org/10.1016/j.carbon.2019.03.017
- Discipline:
- Science